The global hydrogen generation market currently stands at around $160 billion and is expected to grow rapidly, especially if adequate policies are put in place to accelerate the green transition. Clean, and particularly, green hydrogen could become the major driver behind such growth, entirely taking over the hydrogen production landscape by 2050, and reaching a potential market value of over $1.4 trillion.
The idea of hydrogen as an energy source dates back to the 1800s when the Swiss chemist Christian Friedrich Schonbein discovered how to combine hydrogen and oxygen to produce water and electricity (fuel cell effect), a process that was used a few years later to create the first gas battery, known as the Father of Fuel Cells. Today, almost 99% of global hydrogen is used in oil refining and other industries.
Virtually all of hydrogen production and use depends on fossil fuels, consuming over 6% of natural gas and 2% of coal supplies globally. Although some facilities complement production by carbon capture and storage technologies, the generated emissions remain elevated. In 2022, hydrogen production was responsible for around 1,100-1,300 Mt CO2-eq of emissions. Under a net-zero emissions scenario by 2050, the International Energy Agency (IEA) estimates that the average emissions intensity of hydrogen production could drop by half from around 12-13.5 kg CO2-eq in 2022 to 6-7.5 kg CO2-eq per kg of hydrogen in 2030. Moreover, in a recent analysis, Deloitte projects that if adequate policies were put in place and the required investments were mobilized, clean hydrogen development could contribute to cumulative abatements of up to 85 Gt CO2-eq of emissions by 2050.
Despite its immense potential to decarbonize industries, several challenges stand in the way of progress. First, unlike renewables, for which massive deployment through adequate policies and public support has considerably reduced its costs over the past decades, hydrogen cost remains relatively high when compared to other fossil-fuel-based applications. Second, to date, the uncertainty around the outlook of the hydrogen market, as well as the slow progress on the necessary supportive policies, are still limiting the required private investment.
The objective of this research is to study the hydrogen market as a major green energy source, investigating its growth opportunities and challenges across sectors. This research will serve as the first in a series of articles that will study the various top “green” energy sources and their emerging markets.
Executive Summary
1. Enhanced Support from US and Europe: The addition of the US Inflation Reduction Act and the European Green Deal provides substantial financial incentives and policy support, accelerating the development and deployment of hydrogen technologies. These initiatives signify a strong commitment to integrating hydrogen as a crucial component of clean energy strategies.
2. Market Growth and Technological Innovation: The global hydrogen market, valued at approximately $160 billion, is projected to grow significantly, driven by technological advancements in hydrogen production. Clean hydrogen, especially green hydrogen, is expected to dominate this market, highlighting the shift towards sustainable energy sources.
3. Cost and Infrastructure Challenges: Despite the potential, the cost of hydrogen production and the lack of infrastructure remain significant barriers. The research underscores the need for massive investments and international cooperation to overcome these challenges and achieve cost competitiveness with fossil fuels.
4. Sectoral Application and Demand Increase: Hydrogen’s role in decarbonizing various sectors, including transportation, power generation, and industrial applications, is emphasized. The demand for hydrogen is expected to rise substantially, driven by policy adoption and the expansion of hydrogen use into new areas.
5. Need for Comprehensive Policy Frameworks: Achieving the hydrogen economy’s full potential requires coordinated policy efforts, international collaboration, and substantial investments. The research calls for urgent and comprehensive action to support the clean hydrogen value chain, highlighting the importance of policies in stimulating demand and facilitating market growth.
US & European Hydrogen Support
The transition towards a hydrogen economy is receiving significant backing from policy initiatives in both the United States and Europe. These regions are pioneering efforts to integrate hydrogen as a key component of their clean energy strategies, recognizing its potential to significantly reduce carbon emissions across various sectors of the economy. The US Inflation Reduction Act and the European Green Deal are cornerstone policies that highlight the commitment of these regions to fostering the development and deployment of hydrogen technologies.
United States: Inflation Reduction Act
The Inflation Reduction Act (IRA) of the United States represents a historic investment in clean energy and climate action. Within its broad scope, the IRA includes specific provisions aimed at bolstering the hydrogen sector:
- Tax Credits for Clean Hydrogen Production: The IRA introduces a new production tax credit for clean hydrogen (Section 45V), which varies based on the carbon intensity of the hydrogen production process. This incentive is designed to make green hydrogen financially competitive by significantly reducing the cost of production. The tax credit ranges up to $3 per kilogram of clean hydrogen produced, making it one of the most substantial incentives for hydrogen production globally.
- Investment in Hydrogen Infrastructure and R&D: The IRA allocates billions of dollars toward hydrogen research and development, infrastructure projects, and the establishment of regional hydrogen hubs. These hubs aim to accelerate the commercialization of hydrogen technologies and facilitate the development of hydrogen supply chains across the country.
Europe: European Green Deal
The European Green Deal sets an ambitious roadmap for making the EU’s economy sustainable by turning climate and environmental challenges into opportunities across all policy areas. Hydrogen is identified as a critical element in achieving the EU’s decarbonization goals:
- Hydrogen Strategy for a Climate-Neutral Europe: As part of the Green Deal, the European Commission has launched a comprehensive hydrogen strategy to explore the potential of clean hydrogen in contributing to the EU’s goal of achieving climate neutrality by 2050. The strategy emphasizes the role of green hydrogen and aims to install at least 40 GW of renewable hydrogen electrolyzers by 2030.
- Funding and Financial Support: The EU has committed significant financial resources to support the development of hydrogen technologies through the Innovation Fund, the Modernisation Fund, and Horizon Europe. Additionally, the European Clean Hydrogen Alliance brings together industry, governments, and other stakeholders to scale up hydrogen production and use.
Both the US Inflation Reduction Act and the European Green Deal represent critical steps forward in the global effort to harness the potential of hydrogen as a clean energy carrier. By providing substantial financial incentives, policy support, and investment in R&D, these initiatives are laying the groundwork for a sustainable hydrogen economy, demonstrating the pivotal role of government action in accelerating the transition to renewable energy sources.
As you will see, this technology is advancing rapidly. The major issue is adoption, standardization and infrastructure. For hydrogen to truly accelerate, the US or EU needs to choose the direction forward by creating a War-Time Production Act-like decision on production. This would likely be very divisive politically and raise questions of spending priorities. Yet, the way forward is likely to be driven by increasingly violent climate events and insurance companies pulling out of affected areas.
Advances in Hydrogen Production Technology
Hydrogen is the most abundant element in nature; however, it rarely exists in its pure form. Hydrogen typically exists as part of a more complex compound, such as water (H2O) or methane (CH4), which needs to be extracted into pure hydrogen (H2) to be used as an energy source.
Currently, hydrogen can be produced through several available technologies. Different production technologies emit varying intensities of CO2 emissions, with newer and more advanced technologies usually targeting the reduction of emissions in energy production. This largely depends on the underlying fuel or compound from which hydrogen is extracted and the extraction processes used. In particular, hydrogen can be extracted from fossil fuels, such as natural gas or coal, water, or a mix of both. The major hydrogen production technologies:
- Black or brown hydrogen: produced through gasification, where coal or biomass is reacted with high-temperature steam and oxygen in a pressurized gasifier. The process produces synthesis gas – a mix of hydrogen and carbon monoxide – which is then reacted with steam to separate the hydrogen. This is the most polluting hydrogen production technology with 20 kgCO2/kgH2 of emissions released during the process. However, it’s worth noting that ongoing research aims to optimize this process for greater efficiency and lower emissions.
- Gray Hydrogen: produced by reacting natural gas with high-temperature steam, to produce synthesis gas and extract hydrogen. This production technology is the most efficient, cheapest, and most widely used. Although less polluting than brown hydrogen, the process is still carbon intensive, producing around 9kgCO2/kgH2 of emissions. Efforts are underway to implement carbon capture and storage (CCS) technologies to mitigate these emissions.
- Turquoise hydrogen: also relies on natural gas, however through a process of methane pyrolysis rather than gasification. The process produces hydrogen and solid carbon (instead of gaseous) carbon, avoiding the release of CO2 into the atmosphere. Despite its low emission-intensity, the process is expensive and hard to scale to date. Moreover, dealing with upstream methane emissions is still an issue. Research is ongoing to enhance the scalability and cost-effectiveness of this technology, alongside efforts to minimize methane leakage throughout the production process.
- Blue Hydrogen: a similar process to gray hydrogen production, complemented with capture and storage technologies. Blue hydrogen leverages the efficiency of the gray hydrogen production technology, while reducing emissions. Nevertheless, due to upstream methane emissions and residual carbon emissions (the maximum carbon capture rate is currently around 95%), this technology fails to achieve carbon neutrality. Ongoing advancements are focused on improving carbon capture rates and reducing methane leakage to enhance the environmental credentials of blue hydrogen.
- Pink Hydrogen: relies on nuclear power to produce hydrogen through water electrolysis – using an electric current to split water into hydrogen and oxygen. Although low in emissions, the scaling up of nuclear power is the major issue. Research is being conducted to address safety concerns and streamline the nuclear power infrastructure to support the widespread adoption of pink hydrogen.
- Green Hydrogen: like pink hydrogen, green hydrogen is produced through water electrolysis, however using renewable energy (e.g. solar and wind). This is the cleanest and most socially acceptable hydrogen production technology. While still an expensive process, green hydrogen production can easily be scalable and is expected to become more cost-effective as deployment increases, similar to the development of the renewable energy market over the past decade. Continued advancements in renewable energy technologies are expected to drive down the costs associated with green hydrogen production, making it a viable and sustainable alternative for various industrial and energy applications.
Hydrogen Production and Cost
In 2022, global hydrogen production increased by 3% to stand at 95 Mt, of which around 75 Mt were pure hydrogen production, and 20 Mt were mixed with carbon-containing gasses from methanol production and steel production. Natural gas, without capture, utilization and storage (CCUS) technologies, is currently the most common source of hydrogen, accounting for almost two-thirds of hydrogen production per year. This is followed by coal (mostly in China), which accounts for around 21% of production. By-product hydrogen, particularly produced in the petrochemical industry, accounts for around 16%.
To date, low-emission hydrogen production stands at no more than 1% of global output, with the majority coming from fossil fuels that are complemented by CCUS. Water electrolysis, on the other hand, contributes less than 0.1% of global hydrogen production (Figure 1).
Figure 1 – Hydrogen Production by Type of Fuel, 2020 – 2022
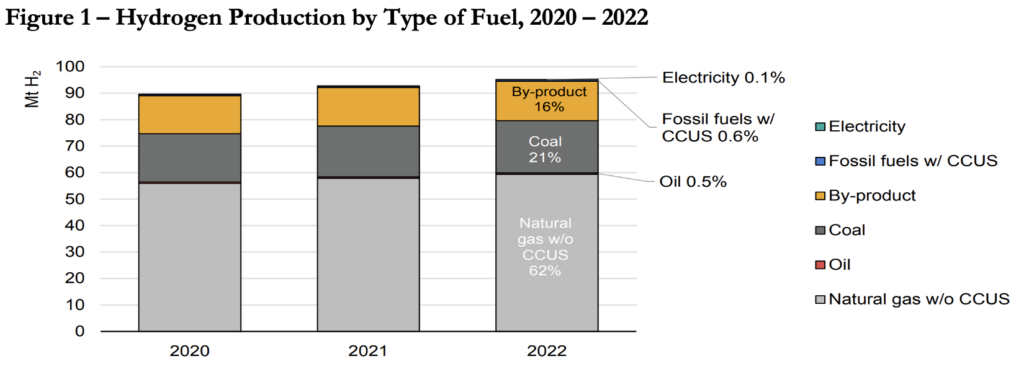
Source: International Energy Agency.
The low percentage of low-emission hydrogen highlights the need for significant advancements in clean energy technologies to mitigate carbon emissions from hydrogen production processes.
Over 70% of global hydrogen production is concentrated in China, the U.S., the Middle East, India and Russia. This owes to the disparity in energy resources and costs across regions, which impacts the cost of producing hydrogen. Almost 45-75% of the cost of hydrogen production depends on the type of fuel used in the process. China is the largest producer of hydrogen, contributing almost 30% of total output, thanks to its abundant coal and large domestic demands for the chemical industry and refineries. On the other hand, natural gas hydrogen is produced at relatively lower costs in the Middle East, followed by the U.S. and Russia, given the abundant and cheaper natural gas there. On the other hand, production costs are higher in countries that import natural gas, such as Europe, Japan, Korea, and India. Understanding the regional disparities in production costs helps policymakers and industry leaders strategize for more efficient and cost-effective hydrogen production.
Although costs can vary per production technology across different regions, natural gas produced hydrogen can be the cheapest globally (ranging from $0.9-3.2 per kg), followed by coal ($1.2-2.2 per kg) and natural gas with CCUS ($1.5-2.9 per kg). On the other hand, hydrogen from renewable electricity remains the costliest to date, in the range of $3-7.5 per kg, depending on the region (Figure 2).
Figure 2 – Hydrogen Production Costs by Fuel
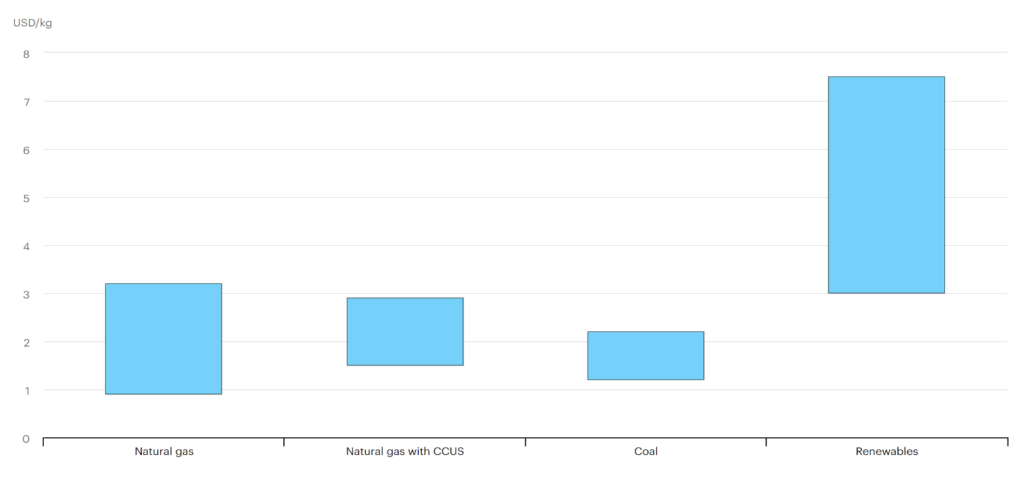
Source: International Energy Agency
These cost differentials highlight the economic challenges and opportunities associated with transitioning to renewable energy sources for hydrogen production.
Nevertheless, with the falling costs of renewable electricity, especially solar and wind in several regions, the market for electrolytic hydrogen is rapidly growing. While water-electrolysis produced hydrogen was no more than 100 kt H2 in 2022, its volume grew by 35% compared to the previous year. Yet, for renewables to become promising for hydrogen production, this would require immensely scaling up the deployment of renewables and dedicating large amounts of the grid electricity to hydrogen production. To put this into context, it is estimated that producing all of today’s dedicated hydrogen supply from electricity would require an electricity demand of 3,600 TWh, which exceeds the EU’s total electricity generation. This highlights the significant challenges in scaling up renewable energy infrastructure to meet the growing demand for green hydrogen and the need for coordinated efforts to overcome these hurdles.
Global Demand for Hydrogen and Potential for Growth
Global hydrogen demand continued to trend upwards, reaching 95 Mt in 2022, a 3% increase over the 2021 level and a continued recovery from the pandemic slump. On the regional level, hydrogen use tends to be concentrated in China (accounting for almost 30%), North America (17%), and the Middle East (13%), followed by Europe and India (which together account for 17%). In 2022, hydrogen demand grew by around 7% in the Middle East and North America and only 0.5% in China. Demand suffered, however, in Europe, due to the slowdown in economic activity, especially in the chemical industry, as well as the surge in the prices of natural gas impacted by the Russia-Ukraine war. These regional trends highlight the importance of geopolitical factors and economic conditions in shaping global hydrogen demand.
To date, virtually all hydrogen (99%) is used in traditional industrial applications, particularly in oil refining, as a feedstock in the chemical industry (to produce ammonia and methanol), and as a reducing agent in the steel industry to produce. On the other hand, less than 1% of hydrogen is currently used in newer applications, such as transport, power generation and storage, or the production of hydrogen-derived fuels (e.g., ammonia or synthetic hydrocarbons). The lack of hydrogen usage in such new areas has stemmed from the fact that end-user technologies are yet to reach commercial maturity, or the lack of hydrogen’s competitiveness compared to fossil fuels or other available low-emissions technologies. Nevertheless, the growing climate agenda and efforts to decarbonize economies are expected to boost hydrogen in untraditional areas, especially where other low-emission technologies are unavailable. This highlights the potential for hydrogen to assume a more substantial role in a future economy that prioritizes decarbonization, provided that technological and economic challenges are effectively addressed.Top of Form
The demand for hydrogen is expected to significantly increase by 2030, however, the extent of the increase will depend on the rate of global policy adoption. The IEA provides three scenarios with different trajectories for hydrogen demand (Figure 3):
- The Stated Policies (STP) Scenario: Under the sectoral policies that are currently in place, hydrogen demand is expected to reach 115 Mt by 2030, representing an annual growth rate of around 2% from the 2022 level. Under this scenario, most of the demand growth would come from traditional uses, with small demand (less than 2 Mt) from new applications or the replacement of unabated fossil fuels in traditional applications. Accordingly, hydrogen would play a small role in achieving climate pledges.
- Announced Pledges (ANP) Scenario: If all climate pledges, Nationally Determined Contributions (NDCs), and net zero targets were fulfilled by governments across the globe, hydrogen demand is projected to reach 130 Mt by 2030, or close to 4% annual growth between 2022-2030. Under such a scenario, around a quarter of hydrogen would be for new applications and the use of low-emission hydrogen in traditional applications. This scenario, however, would assume that governments step up their policy action to stimulate hydrogen demand worldwide, and especially clean hydrogen.
- Net Zero Emissions (NZE) Scenario: This is the most ambitious scenario, which assumes that net zero emissions are met by 2050, requiring even further policy action and commitments than are currently in place. Under this scenario, demand would grow by an annual growth rate of around 6% up to 2030, to reach over 150 Mt of hydrogen, of which 40% would be for new applications.
These scenarios emphasize the crucial importance of policy frameworks and international cooperation in facilitating the transition to a hydrogen-based economy and attaining global climate objective
Figure 3 – The Evolution of Hydrogen Demand, 2019-2030
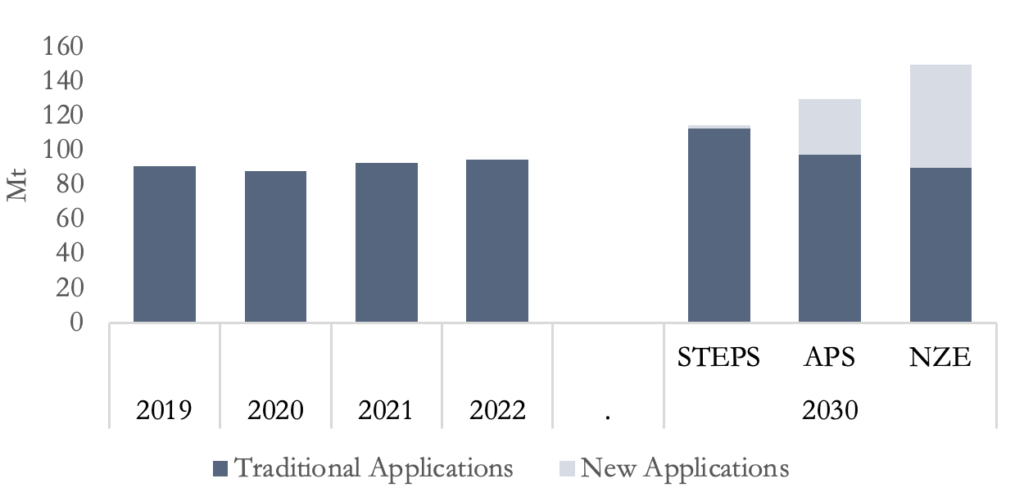
Source: International Energy Agency, 2022, 2023
Hydrogen Usage and Opportunities by Sector
Hydrogen Use in Oil Refining
In 2022, oil refining accounted for almost 41 Mt (43%) of hydrogen demand, primarily used to turn crude oil into several end-user products, through hydrotreating (to remove impurities such as sulfur from crude oil) and hydrocracking (to upgrade heavier oil into higher-value products). Almost 80% of the global hydrogen used in refineries is produced on site, as a byproduct of the naphtha reforming process or through dedicated production, while 20% is sourced from merchants.
Most of the hydrogen used in refining relies on natural gas for its production, with minimal supplies (especially in China and India) depending on coal. Accordingly, the cost of hydrogen in oil refining can vary by region, depending on the price of fuel. For example, in the U.S., hydrogen costs around $0.7 per barrel of oil refined (due to the relatively lower cost of natural gas), compared to over $1 and almost $2 in the EU and Asia, respectively. Given the highly competitive nature of the oil refining market, even the smallest cost advantages could noticeably impact the profit margins (Figure 4).
Figure 4 – Hydrogen Production Costs Compared to Refining Margins, 2018
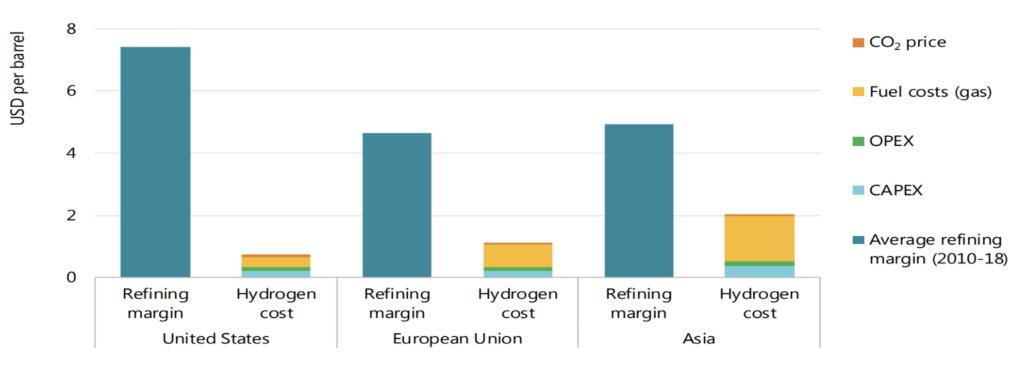
Source: International Energy Agency
Moreover, recent advancements in carbon capture and storage (CCUS) technologies have enabled a more environmentally sustainable production of hydrogen, mitigating concerns over emissions associated with hydrogen production.
Supported by the growth in refining activity and the increasing requirements for hydrotreating and hydrocracking, the demand for refinery hydrogen has grown significantly in recent years and is expected to grow further in the near term. In recent years, to reduce air pollution, regulations that limit the acceptable amount of sulfur allowed in fuels have been implemented in several countries. For example, a global cap of 0.5% (from a previous 3.5%) sulfur content in marine fuels was applied by the International Maritime Organization (IMO) in 2020. Moreover, several countries such as China already have requirements to reduce sulfur content in road transport fuel to less than 0.0015%. This trend is expected to substantially increase hydrogen demand in the oil refining sector.
The increasing emphasis on environmental sustainability and the transition to cleaner energy sources are expected to drive further investments in low-emission hydrogen production technologies, particularly electrolysis powered by renewable energy sources.
However, the increasing share of US oil production has led to a shift towards sweeter crude oil varieties with lower impurities, which has partially reduced the necessity for hydrogen in hydrotreating. Additionally, as climate initiatives progress and the demand for oil products decreases, the demand for refinery hydrogen may slow down.
Overall, hydrogen demand for oil refining is expected to follow different trajectories based on the pace of climate policy action. Under the STP scenario, refinery hydrogen demand is expected to increase substantially to around 47 Mt by 2030, while under the ANP scenario, it is expected to only increase modestly to 43 Mt. The latter would account for higher climate action and increased use of cleaner technologies and fuels, such as electrification, hydrogen, and hydrogen-derived fuels, in the transport sector.
Both scenarios do not envision remarkable increases in the use of low-emission hydrogen in refining, owing to the limited number of announced projects that aim to produce low-emission hydrogen in refineries. It is estimated that if all those projects are to be realized by 2030, only 1.3 Mt of low-emission hydrogen would be used in refineries, the majority of which (1.1 Mt) would be from fossil fuels with CCUS, while only 0.2 Mt from electrolysis. On the other hand, under the NZE scenario, to successfully reach net zero by 2050, the trend for hydrogen use in oil refining would have to reverse, decreasing to 35 Mt by 2030, 15% of which would be met by low-emission hydrogen (Figure 5).
Figure 5 – The Evolution of Hydrogen Demand in Oil Refining, 2019-2030
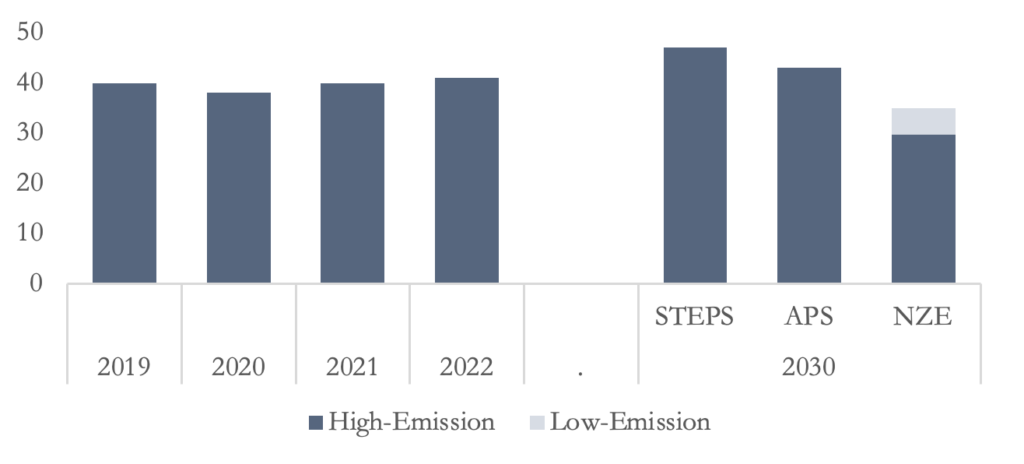
Source: International Energy Agency, 2022, 2023
The Potential for low-Emission Hydrogen in Oil Refining
In 2022, the production of hydrogen for oil refining resulted in almost 240-380 Mt of CO2 emissions, close to a quarter of total refinery emissions. As the demand for refinery hydrogen increases, the growth in emissions will depend on the fuel used to produce it. To date, less than 1% of the hydrogen used in refining is produced using low-emission technologies. However, the emergence of advanced low-emission hydrogen production methods, such as electrolysis powered by renewable energy sources, presents promising avenues to mitigate emissions stemming from hydrogen production in refineries.
On the positive side, the switch to low-emission hydrogen in oil refining can provide a significant opportunity to support the overall development and growth of the clean hydrogen market. Switching to low-emission hydrogen in refineries is a straightforward process, as it involves a like-for-like substitution rather than a fuel switch. This, coupled with the ample demand, makes the refining industry a potential early market for clean hydrogen. The culprit, however, is the relatively higher cost of low-emission hydrogen and limited policy action to promote its adoption. Effective implementation of incentives and regulations by policymakers is crucial to drive the widespread adoption of low-emission hydrogen technologies in refineries, thereby accelerating the transition toward a more sustainable energy landscape.
Reducing emissions from hydrogen use in industry requires first, reducing the overall demand for oil products and second, increasing the use of low-emission hydrogen in refineries. Two paths exist to increase the use of low-emission hydrogen: (1) complementing fossil fuel hydrogen with CCUS, or (2) using electrolysis to produce clean hydrogen. The former option offers a more competitive and easier implementable pathway, however, without adequate policies to incentivize its use, progress could be slow. On average, the introduction of CCUS adds a cost of around US$ 0.25-0.5 per barrel of oil refined, which is higher than the current carbon price (US$ 0-0.1 per barrel). As a result, it is more cost effective for refineries to pay for their CO2 emissions than to invest in its capture and storage. Policies that increase the cost of carbon as well as reduce the cost of storage and increase its availability are hence essential for such matters. According to the IEA, a carbon price of at least US$ 50/tCO2 is needed to make the deployment of CCUS with natural gas an economically attractive option.
Furthermore, investments in research and development are paramount to enhancing the efficiency and reducing the cost of electrolysis technology, thereby facilitating the upscale production of low-emission hydrogen in refineries.
While electrolytic hydrogen is not currently utilized in refineries, potential avenues for its adoption are emerging, particularly in regions like Germany and the Netherlands. Notable instances include Shell’s 10-MW electrolyzer facility at its Rheinland refinery in Germany, with prospects for expansion to a capacity of 100 MW.
Hydrogen Use in Industry
Currently, most of the hydrogen used for industrial purposes, other than oil refining, is concentrated in the chemical industry (for the production of ammonia and methanol) and in the steel industry (for the production of direct reduced iron (DRI)), with very small amounts used in other special industries (e.g., glass making and electronics). In 2022, the chemical and steel industries accounted for 53 Mt (56%) of hydrogen demand, of which 60% was for ammonia production, 30% for methanol production, and 10% for the production of DRI.
Almost all the hydrogen used in industry is produced on site, in the industrial facility, from fossil fuels. For example, in the chemical industry, about 65% of the hydrogen used to produce ammonia and methanol is from natural gas, 30% from coal (mostly in China), and a minimal amount is from oil. As a result, industrial hydrogen production is a high-emitting process, with substantial potential for emission reduction. In 2022, the production of hydrogen for industrial use resulted in almost 680 Mt of CO2 emissions, equivalent to a little less than 10% of industrial emissions.
Given the current trends and announced policies, demand for hydrogen-derived products, and hence industrial hydrogen, is expected to continue increasing over the medium term. Under the current demand trends, hydrogen for industrial use is projected to increase by 3% annually, to reach around 65 Mt by 2030. New hydrogen applications, in particular, have great potential to accelerate the climate agenda and reduce emissions. Those can include advanced low-emission production technologies to produce traditional products (e.g., using electrolysis or installing CCUS to produce ammonia, methanol, or DRI), the production of 100%-hydrogen DRI, or the use of hydrogen for industrial heat. Under the ANP scenario, where governments would fulfill their announced commitments, new applications for industrial hydrogen would grow to constitute close to 20% of demand by 2030. Under a net-zero scenario by 2050 scenario, however, more aggressive policies to stimulate demand for clean hydrogen would be required to achieve the target, where hydrogen demand would rise by 4% annually, to reach 70 Mt by 2030, of which at least a third would be low-emission hydrogen and 16% utilized in non-traditional applications (Figure 6). In addition, it’s crucial to note that the transition to cleaner hydrogen production methods is essential for achieving emission reduction targets and sustainable industrial growth.
Figure 6 – The Evolution of Hydrogen Demand in Industry, 2021-2030
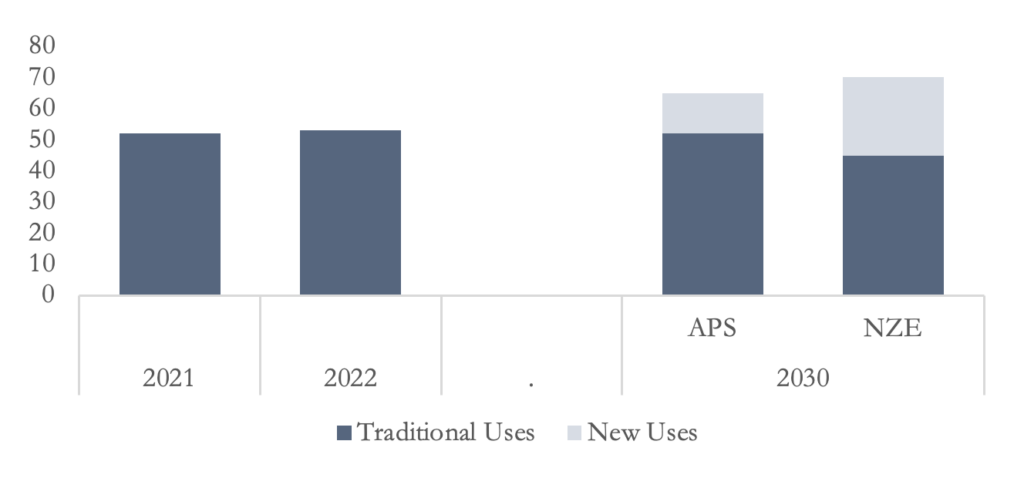
Source: International Energy Agency, 2022, 2023
While it is evident that the demand for hydrogen in the industrial sector will continue to increase, the question is whether this demand can be met while reducing emissions. To date, the use of low-emission industrial hydrogen accounts for no more than 0.5% (285 Kt), most of which depends on fossil fuels with CCUS. Two main potential pathways exist to reduce emission from industrial hydrogen, the use of CCUS and using electrolytic hydrogen. As discussed before, those technologies would require that sufficient CO2 transport and storage infrastructure are available, that carbon pricing is economical to shift incentives, and that renewables are adequately scaled up to ensure enough electricity supply. In most regions, the use of CCUS is currently the more cost-effective choice. However, as the price of renewable energy continues to trend downwards, electrolysis might gain an edge.
The Potential for low-Emission Hydrogen Use in the Chemical Industry
Whether CCUS or electrolysis is used to reduce emissions from hydrogen in the chemical industry, both pathways would create considerable demand growth for energy inputs, in particular natural gas or electricity. Under a future scenario where, by 2030, all hydrogen demand for ammonia and methanol would use CCUS-equipped hydrogen, around 323 bcm of natural gas would be needed (equivalent to a tenth of current global natural gas demand). Yet, close to 450 MtCO2 would need to be captured per year. To put this into context, the largest CCUS installation today can capture a maximum of around 1 MtCO2 a year. Reaching this goal would require building around 4 new CCUS projects per month up to 2030, to install close to 450 new projects of the current capacity by then (Figure 7).
Figure 7 – The Implications of Cleaner Process Routes for Methanol and Ammonia Production
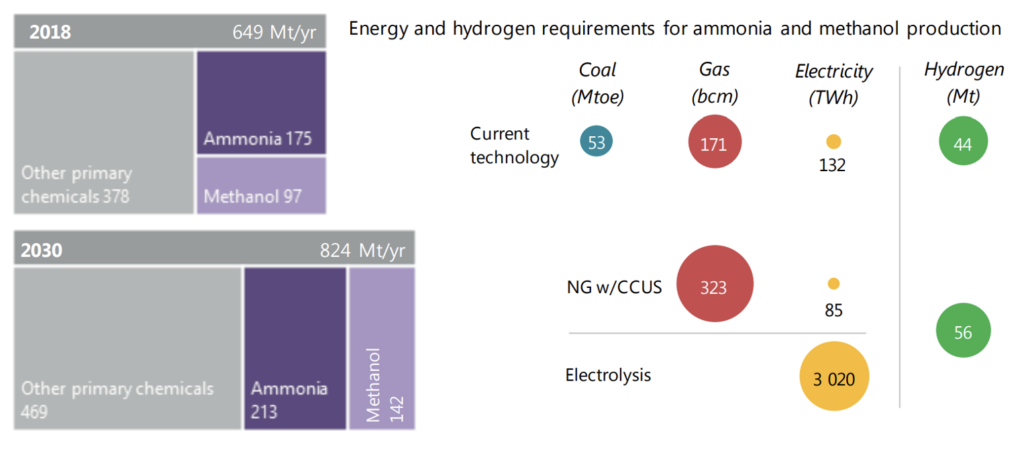
Source: International Energy Agency
Achieving this level of decarbonization would necessitate significant investment and coordination among industries, governments, and stakeholders to overcome logistical, technical, and financial challenges.
On the other hand, if future ammonia and methanol were to be entirely produced from electrolytic hydrogen by 2030, around an additional 3,000 TWh of renewable electricity would be required per year (equivalent to around 11% of the current global production). In addition, around 350-450 GW of electrolyzer capacity would be required, which, given the 100+ MW scale that currently exists, necessitates close to 6 installations of such size per week up to 2030, to reach 3,500-4,000 installations by 2030.
This transition to electrolytic hydrogen production would require a monumental scaling up of renewable energy infrastructure, posing significant logistical and technological challenges.
The key factor that will determine the widespread use of low-emission hydrogen in the chemical industry is cost, particularly from natural gas and electricity prices. To date, using cleaner hydrogen to produce ammonia and methanol is more expensive than commercially available high-emission hydrogen (without CCUS). Depending on the relative costs of fossil fuels and electricity in different regions, production costs can vary widely. For example, in regions, where renewable energy is cheaper (e.g. Chile, China, or Morocco), electrolytic hydrogen might hold great potential. Many of those locations, however, are far from the demand centers for industrial hydrogen, and adding the storage and transportation costs of hydrogen and the required infrastructure might degrade its cost benefits. It is estimated that at electricity prices below USD 15-50/MWh for ammonia and USD 10-65/MWh for methanol, hydrolytic hydrogen is expected to become more competitive than CCUS-equipped natural gas. Yet, only at prices below USD 10-40/MWh for ammonia and USD 5-50/MWh for methanol would electricity be able to compete with the current natural gas without CCUS technologies in place.
The Potential for low-Emission Hydrogen Use in the Steel Industry
In the steel industry, emissions can be reduced through using low-emission hydrogen in the traditional production process of DRI, as well as replacing the natural gas that is directly consumed in the production of DRI, by pure hydrogen from electrolysis. Under a pathway that aligns the sector with the goals of the Paris Agreement, it is estimated that 30% of the natural gas used in the production of DRI would be directly replaced by clean hydrogen. This ambitious transformation would require 230 TWh per year of renewable electricity (equivalent to Turkey’s current electricity consumption). Still, natural gas would continue to play a role in supplying the remaining hydrogen, with a required 31 bcm per year (equivalent to Spain’s current natural gas consumption). Those estimates are presented in Figure 8.
Figure 8 – Energy Implication of Fulfilling Hydrogen Demand via the DRI Route
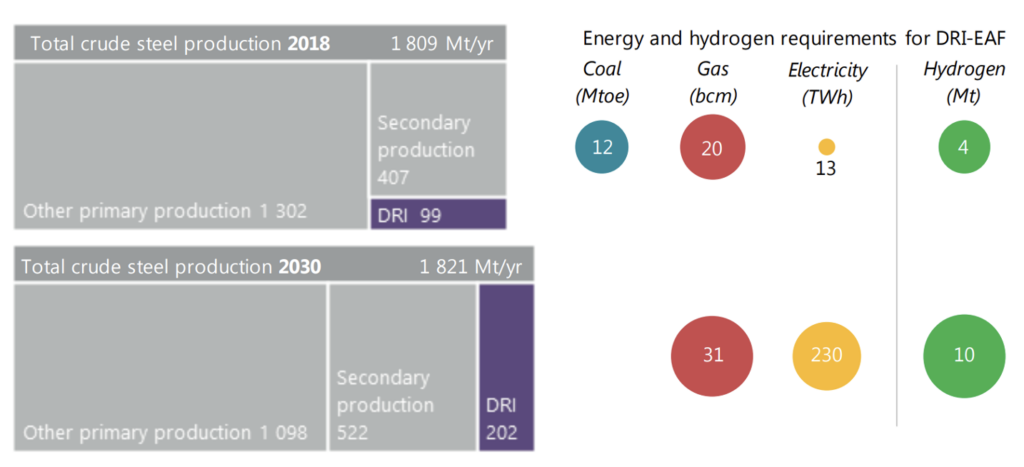
Source: International Energy Agency.
Transitioning the steel industry to low-emission hydrogen presents a complex challenge, as it involves significant infrastructural changes and technological advancements across the entire supply chain, from production to distribution.
In the longer term beyond 2030, however, greater ambition would be required to reduce emissions from the steel industry. In particular, more electrolytic hydrogen would be required to produce 100%-hydrogen DRI and to reach a 100% hydrogen-based pathway. For this to happen, over a massive 2,500 TWh of renewable electricity is required to produce hydrogen per year, which is equivalent to the current consumption of India, Japan, and Korea together.
Such a transformation would necessitate unprecedented levels of investment, innovation, and collaboration among stakeholders to overcome technical, economic, and logistical barriers.
From a cost perspective, for electrolytic hydrogen to be cost competitive into the long-term 100% hydrogen-based scenario, it is estimated that renewable electricity prices would need to drop below USD 5–35/MWh to win over natural gas with CCUS, and even lower to win over the current natural gas without CCUS technologies.
Hydrogen Use in Transportation
The use of hydrogen in the transport sector remains very limited, standing at around 32 Kt in 2022, representing only 0.03% of total hydrogen demand and around 0.003% of total transport energy. However, transport hydrogen demand has been growing at a remarkable pace, expanding by around 45% in 2022.
Most of the hydrogen demand in the sector is used in road transport, through fuel cell electric vehicles (FCEVs). More recently, though, hydrogen consumption has started witnessing some demand from the rail sector and fuel cell ferries. Moreover, if technologies for ammonia- and methanol-ready vessels reach commercial maturity in the coming years, demand could increase for hydrogen use in the shipping sector.
Additionally, hydrogen-powered drones and aircraft are emerging as potential applications in the aviation sector, promising a cleaner alternative to conventional fossil fuel-powered aircraft.
FCEVs provide the largest demand for hydrogen in the transport sector. By June 2023, the global stock of FCEVs reached around 78,000 vehicles. Almost 81% or 63,000 of the fleet were cars and vans, mostly sold in Korea (55%) and the U.S. (28%). Heavy-duty trucks and buses, on the other hand, represented around 10% (8,000 vehicles) and 9% (7,000 vehicles) of total FCEVs in June 2023, respectively. China remained the largest market for fuel cell trucks and buses, for 95% and 85% of the global vehicles, respectively.
The use of hydrogen and hydrogen-based fuels can play an important role in decarbonizing the transportation sector, being the only vehicles besides battery electric vehicles (BEVs) that produce no emissions. The major obstacle to their wider adoption, however, pertains to their low cost-efficiency. Practically, any vehicle can operate on hydrogen or hydrogen-derived fuels in internal combustion engines, which could create a huge demand for transport hydrogen. If all current global vehicles (1 billion cars, 190 million trucks and 25 million buses) were to become FCEVs, hydrogen demand would increase by more than 4 times, reaching 200 MtH2 per year. Up to 2030, the potential for transport hydrogen demand could even be larger, given that demand for trucks and passenger cars is projected to grow further over the coming decade.
Moreover, hydrogen-powered trains are also being explored as an eco-friendly alternative in railway transportation, offering zero-emission solutions for both passenger and freight services.
Nevertheless, despite the huge potential for hydrogen in road transport and the environmental advantages that this could offer, actual deployment would depend largely on vehicle and fuel costs, as well as the policies in place to boost their demand and production.
A very important factor that impacts the cost of hydrogen fuel for transportation is the utilization of hydrogen refueling stations (HRS). In 2022, the number of installed HRSs worldwide exceeded 1,000, a remarkable expansion from the 700 stations in 2021 and less than 400 stations in 2018. The number of refueling stations is expected to pick up momentum even further to meet the rising demand for FCEVs. Nevertheless, the utilization ratio of FCEVs to fueling stations remains low, at a global average of 80 vehicles per station, which reduces the cost-effectiveness of hydrogen fuel for road transport. For example, a ratio of around 10 vehicles per station (close to that in France), would mean that for a small station of 50 kgH2, pumps are utilized at a capacity of only 10%, making the delivery of hydrogen inefficient. Improving the efficiency and accessibility of hydrogen refueling infrastructure will be critical in supporting the widespread adoption of hydrogen-powered vehicles.Figure 9 shows the number of HRS and their utilization across countries.
Figure 9 – Hydrogen Refueling Stations and FCEVs Utilization
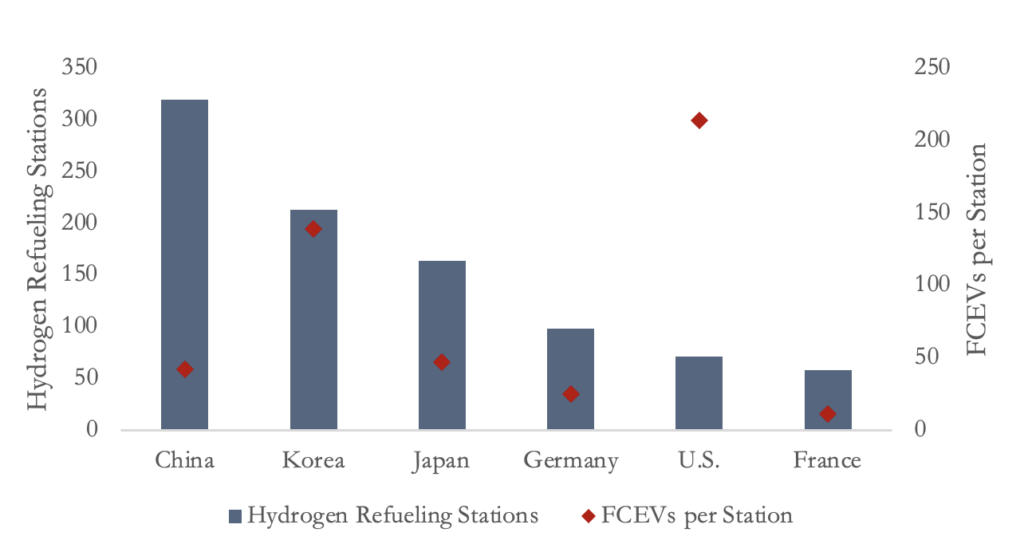
Source: Remzi Can Samsun and Michael Rex (2023), Deployment of Fuel Cell Vehicles in Road Transport and the Expansion of the Hydrogen Refueling Station Network: 2023 Update
Hydrogen Use in Energy Generation
Although technologies for the use of pure hydrogen in electricity generation (e.g., fuel cells and internal combustion engines) do exist, hydrogen represents less than 0.2% of the global electricity generation mix, most of which is not in the form of pure hydrogen but linked to the use of gasses in oil refining and industry. The potential of hydrogen in the power sector includes the possible cofiring of hydrogen-based fuels (e.g. ammonia) in traditionally coal-fired plants to reduce emissions, the use of hydrogen or ammonia as fuels in gas turbines to offer flexibility in electricity systems, as well as using hydrogen-based fuels for large-scale and long-term energy storage to balance seasonal variations in electricity demand or variable renewable power generation. Hydrogen offers promising potential as a clean energy carrier in the power sector, contributing to decarbonization efforts and enhancing grid stability.
According to IEA, the announced projects that use hydrogen and ammonia in the energy sector could potentially raise the installed capacity by 65% between 2022 and 2030, to generate around 5,800 MW of power by 2030. Almost 70% of this capacity would be linked to the use of hydrogen-based fuel gas turbines, while 10% would be used in fuel cells and 3% to cofire ammonia in coal-fired power plants. Regionally, around 40% of those projects would be in the Asia-Pacific region, while 25-35% would be in Europe and North America. Needless to say, however, while this would help reduce the carbon footprint of the energy sector, the economic viability of substituting ammonia for coal would depend in the first place on the availability of low-cost ammonia and thus on the development of hydrogen use in the chemical industry.
In addition, hydrogen and ammonia could be used directly or through fuel cells in gas turbines to offer flexible generation technologies for load balancing and peak load generation. The competitiveness of this technology against natural gas-fired generation, however, would depend on the price of natural gas as well as carbon prices. For example, with a load factor of 15% and gas price of $7/MMBtu, a carbon price of at least $100/tCO2 and a hydrogen price of USD 1.5/kgH2 would be needed to make hydrogen-fired electricity as competitive as natural gas. At a hydrogen price of $2/kg H2, a carbon price of at least $175/tCO2 would be needed.
Finally, hydrogen can offer great potential for long-term energy storage to balance the demand and supply of energy, as well as facilitate the import of hydrogen-based fuels. Several storage technologies exist, including pumped-hydro, battery, and compressed hydrogen and ammonia storage, which vary in their efficiencies and capital costs. For short discharge durations below a few hours, pumped-hydro and battery storage tend to be more competitive options due to their lower capital costs. For longer durations, compressed hydrogen and ammonia become more attractive. In general, for discharge durations over 20-45 hours, compressed hydrogen tends to be the most economical choice.
Hydrogen Use in Buildings
The demand for hydrogen in buildings, particularly for heating and power generation, provides the lowest demand among all sectors. Demand is currently negligible, and under the current policies, is expected to reach no more than 0.03 Mt by 2030. Even under the NZE scenario, demand is unlikely to exceed 1 Mt by 2030, accounting for around 0.14% of total energy demand in the sector. Exploring hydrogen applications in buildings, especially for heating purposes, could contribute to reducing carbon emissions and improving air quality in urban areas.
Although hydrogen use in buildings can offer some new niche applications such as heating, especially in old and poorly insulated buildings, the culprit remains the low efficiency level and high level of energy loss that is associated with hydrogen conversion, transport and use in buildings. Without new and repurposed infrastructure, the buildings sector is not expected to provide a major growth driver for the hydrogen market.
The Economics of Clean Hydrogen: Huge Potential with Several Challenges
The value of global hydrogen generation stood at around US$160 billion in 2023, with 11% growth over its value in 2022. The market is projected to rapidly expand over the next three decades, with clean hydrogen in particular reshaping the global energy map. According to a recent study by Deloitte, the global hydrogen market is estimated to expand by a cumulative annual growth rate (CAGR) of 22%, reaching a value of $642 up to 2030, then growing by a further 4% CAGR to hit US$1.4 trillion by 2050 (Figure 10). The escalating value of the global hydrogen market highlights its potential to revolutionize the energy sector and drive substantial economic development.
Figure 10 – The Evolution of the Global Hydrogen Market Value, 2022-2050
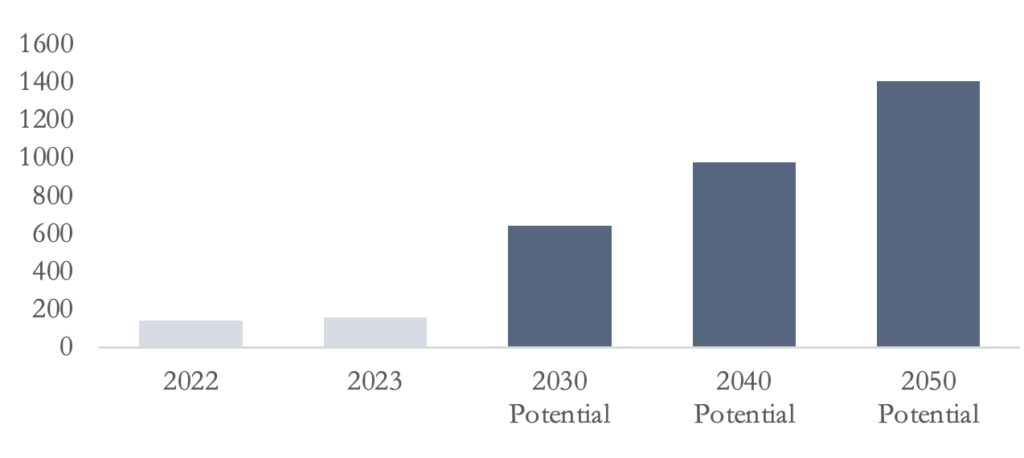
Source: Market-and-Markets Research and Deloitte.
Deloitte’s analysis suggests that by 2050, clean hydrogen could stand on its own in the supply mix. To date, cost remains the major obstacle preventing the rapid uptake of clean hydrogen technologies. Nevertheless, as with the renewable electricity market that saw production costs dramatically fall with public support and mass deployment, economies of scale can support the clean hydrogen market. With the right policies in place, it is estimated that clean hydrogen technologies could break even with fossil-fuel-generated hydrogen by 2023, for pure hydrogen and ammonia, and before 2050, for methanol and sustainable aviation fuels. Under such a scenario, green hydrogen is estimated to dominate the mix of clean hydrogen. As the cost of renewable electricity continues to drop, green hydrogen is expected to grow by an average annual rate of 7.7% in the two decades leading up to 2050, to account for 40% of the total mix in 2030 and over 60% by 2050 (with the remaining coming from blue hydrogen). The transition to clean hydrogen technologies hinges on supportive policies and the realization of economies of scale.
The rapid scaleup and falling cost of renewable energy across the World can facilitate the production of green hydrogen in most countries, provided that adequate government policies and buildup of infrastructure are in place. However, developing countries are expected to gain a comparative advantage by 2050, pertaining to their relatively lower costs of production. For example, in 2050 the cost of green hydrogen production in Europe is estimated to be 4 times that in North Africa. Countries such as Australia, Chile, Mexico and those in sub-Saharan Africa, the Middle East, and North Africa especially have the potential to become major exporters of green hydrogen to the rest of the world.
The growing demand for hydrogen across sectors and new applications is expected to be the main driver behind this remarkable expansion. By 2050, clean hydrogen will mostly be used to decarbonize the steel, chemical, and other industries (42% of demand), the transport sector including in FCEVs, shipping, and aviation (36%), the energy sector for energy storage and flexibility (20%). The use in buildings will remain minimal at no more than 1%. While it is expected for all major economies seeking to decarbonize their systems to boost their uptake of clean hydrogen by 2050, around 80% of the demand will come from China (23%), North America (17%), Europe (16%), India (13%), and the Middle East and North Africa (8%).
By 2050, clean hydrogen trade is expected to generate around $280 billion of revenues for exporters, becoming an important driver of economic growth. In particular, 80% of the export market will be dominated by North Africa (40%), North America (23%), Australia (14%), and the Middle East (7%).
Achieving Goals Requires Massive Investment
According to Deloitte’s analysis, to achieve those ambitious targets, a massive $9.4 trillion investment would need to flow into the global hydrogen value chain, with about a third going to developing countries (Figure 11). To put this into context, in 2022, the global investment in the oil and gas industry stood at around $0.41 trillion. So, while the figure seems huge, the cumulative amount of investment needed in green hydrogen is equivalent to 23 times the annual investment in oil and gas, and it needs to be raised over a 27-year period. Almost 75%, or $7.2 trillion, of this amount, would need to be in green hydrogen production, which could pose industrial and deployment challenges, especially as it would require massively scaling up renewable energy assets. In particular, capital expenditure would be needed in power generation (with around $4.6 trillion dedicated to the manufacturing and installation of additional solar and wind capacities) and electrolyzers ($2.6 trillion).
Figure 11 – $9.4 Trillion Needed Cumulative Investments in the Hydrogen Value Chain by 2050
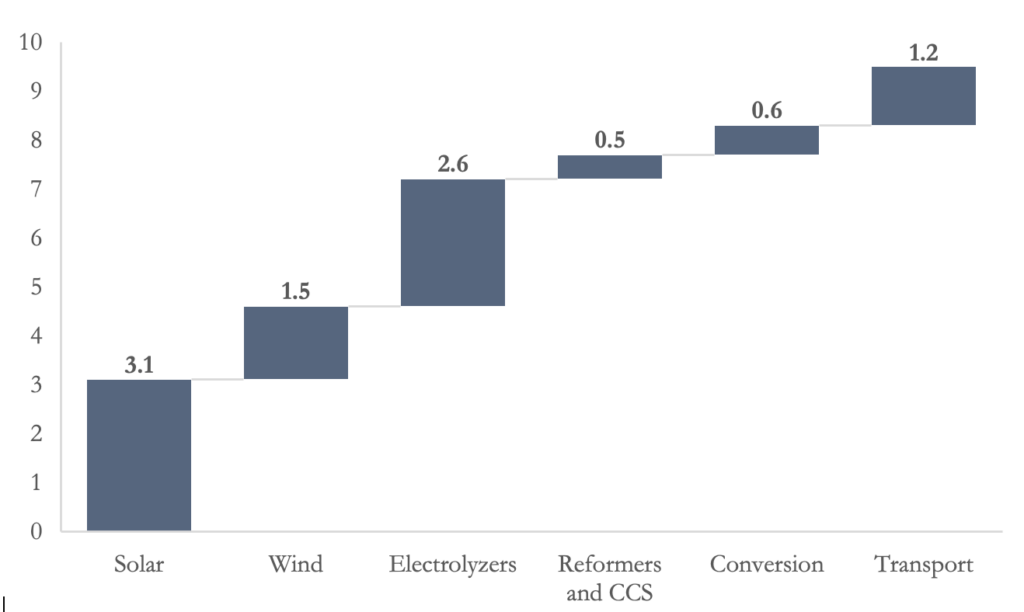
Source: Deloitte.
Adequate Policies Will be Critical to Ramp Up the Clean Hydrogen Value Chain
For this to become manageable, adequate policies to incentivize green technologies and reduce spending on oil and gas must step up. The high cost, lack of infrastructure, and the need to scale up renewable energy sources to power green hydrogen continue to provide investment challenges. For hydrogen to realize its full potential, adequate funding and investment in production and infrastructure is essential. Unfortunately, the uncertainties surrounding the market outlook of clean hydrogen, including the uptake of demand, still deter private investments and prevent the necessary economies of scale from developing. It is thus crucial for governments worldwide to steer their policies in a manner that would boost green hydrogen uptake and give more visibility on the potential of the market.
Some countries have started putting in place targeted policies to support the shift to clean hydrogen use. The US Inflation Reduction Act is a case in point for such policies, where the tax credit of up to US$3/kg for green hydrogen and US$1/kg for blue hydrogen more than closes the cost gap with fossil-fuel-based technologies. Moreover, in 2020, the EU and Norway launched the Important Projects of Common European Interest (IPCEI) program to support private investment in the green hydrogen value chain by offering direct subsidies and public financing. However, more global policy actions need to rapidly adjust if we were to reach the required targets on time.
First, governments across the globe must adopt stringent climate regulations, such as carbon pricing, carbon contracts for differences, green fuel usage quotas, and green fuel standards. Moreover, those policies need to include clear and well-managed timelines and milestones for the development of the clean hydrogen economy, including electrolysis capacity and the needed investments in renewables. These efforts would be crucial for setting the stage and anchoring investors’ expectations, creating appetite, and encouraging the shift from fossil fuels to clean hydrogen technologies. To the IEA, a total of 41 governments, which account for close to 80% of global energy-related CO2 emissions, have released their hydrogen strategies. Nevertheless, faster action is needed to create demand for and accelerate investments in low-emission hydrogen.
Second, governments worldwide need to intensify their subsidy efforts to reduce the costs of uptake of clean hydrogen, supporting the market in its initial stages. According to BloombergNEF, progress is being made, yet, more is needed for hydrogen to offer a promising path to decarbonization. The cost of electrolytic green hydrogen has fallen by almost 40% in the five years leading up to 2020 and is expected to decrease even further if deployment momentum picks up. This would require cumulative subsidies of at least $150 billion to 2030, to reduce the cost of clean hydrogen to $2/kg (comparable to $15/MMBtu for fossil fuels) by 2030 and $1/kg ($7.4/MMBtu) by 2050 (Figure 12).
Top of Form
Figure 12 – The Economics of Hydrogen Economy – Needed Subsidies to 2030

Source: BloombergNEF
Finally…!
The journey towards harnessing the power of hydrogen as a clean energy source across various sectors presents both immense opportunities and significant challenges. While technological advancements and supportive policies pave the way for its widespread adoption, obstacles such as high production costs, infrastructure limitations, and the need for policy frameworks persist.
The global hydrogen market has grown considerably in recent years, primarily driven by global energy demand trends rather than hydrogen-specific policies. Despite this growth, current hydrogen use remains concentrated in traditional applications such as oil refining, steel, and chemical industries, leaving untapped potential in sectors like transportation and power.
To date, the majority of hydrogen production relies on fossil fuel energy, resulting in substantial CO2 emissions. However, the emerging market for clean hydrogen, especially green hydrogen, offers significant potential for emissions reduction and transition to sustainability. Cost projections suggest that clean hydrogen could compete with fossil fuels by 2030 and become the predominant hydrogen technology by 2050, provided there is global policy action to accelerate the green transition and incentivize private investments across the hydrogen value chain.
While some countries have made progress in this regard, faster and more comprehensive action is needed to realize the full potential of clean hydrogen. International collaboration and investment are crucial for driving this transition forward, enabling the global community to unlock the full potential of hydrogen as a key enabler of the low-carbon energy transition. By addressing challenges through collaboration and investment, the world can pave the way towards a sustainable future powered by hydrogen.